Research
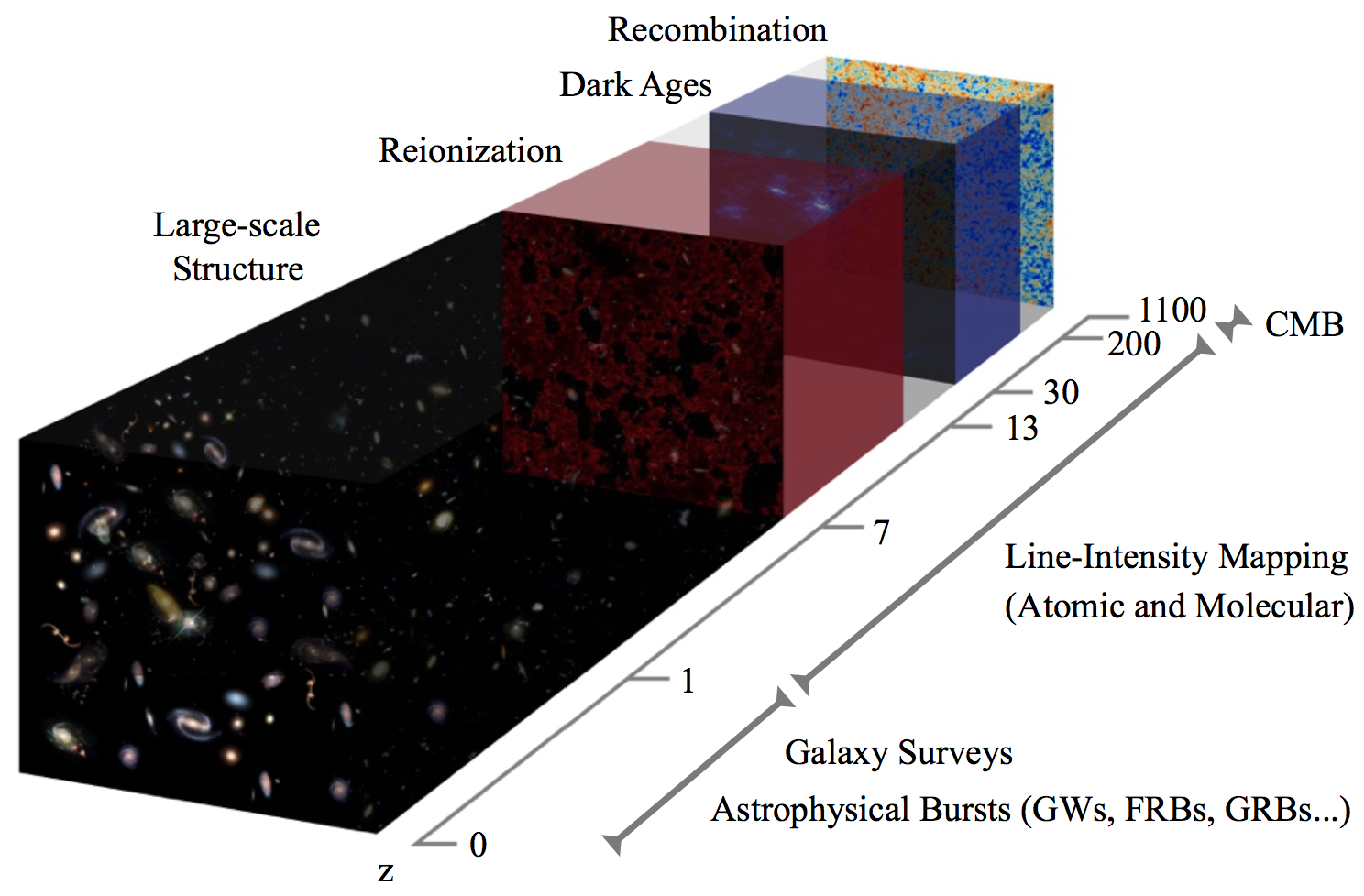
The Observable Universe: probes of different epochs in its history.
Opening Up New Windows to Study the Universe
The Standard Model of Cosmology aims to explains the cosmic
evolution from a fraction of a second after the Big-Bang
singularity to the current period of accelerated expansion
with only a handful of parameters. Over the past decade, it
has withstood a wide series of observational tests. Yet
several gaping holes remain in the theory:
- How did inflation begin and come to an end?
- What makes up the dark matter in the Universe?
- What is the nature of dark energy?
- How did galaxies and clusters of galaxies form and evolve
to make up the large scale structure we observe today?
Going forward, we must develop new ways to probe these
fundamental questions by accessing the full volume of the
observable Universe. This is the focus of my research.
The Cosmic Microwave Background: Exploring the Early Universe
Line-Intensity Mapping: Astrophysics and Cosmology at High Redshifts
Gravitational waves from Mergers of Compact Objects: a Powerful New Probe
Why am I interested?
Gravitational waves from Mergers of Compact Objects
Gravitational wave signals open up a new window to study the Universe. Black hole mergers in particular are uniquely useful in testing both cosmological models and astrophysical processes. I have thoroughly investigated the relation between gravitational waves and compact dark matter and am working on probing dark energy, as well as stellar-black-hole formation, globular clusters and formation of supermassive black holes.
Primordial Black Hole Dark Matter
How would we detect PBH dark matter?
As illustrated in the figure below (from Kovetz, PRL 2017), we can use an analogy with the detection methods of particle dark matter. Direct detection looks for signatures of direct interactions between standard astrophysical objects and PBHs. Indirect detection examines the signal from “annihilation” of two PBHs, i.e. gravitational waves.
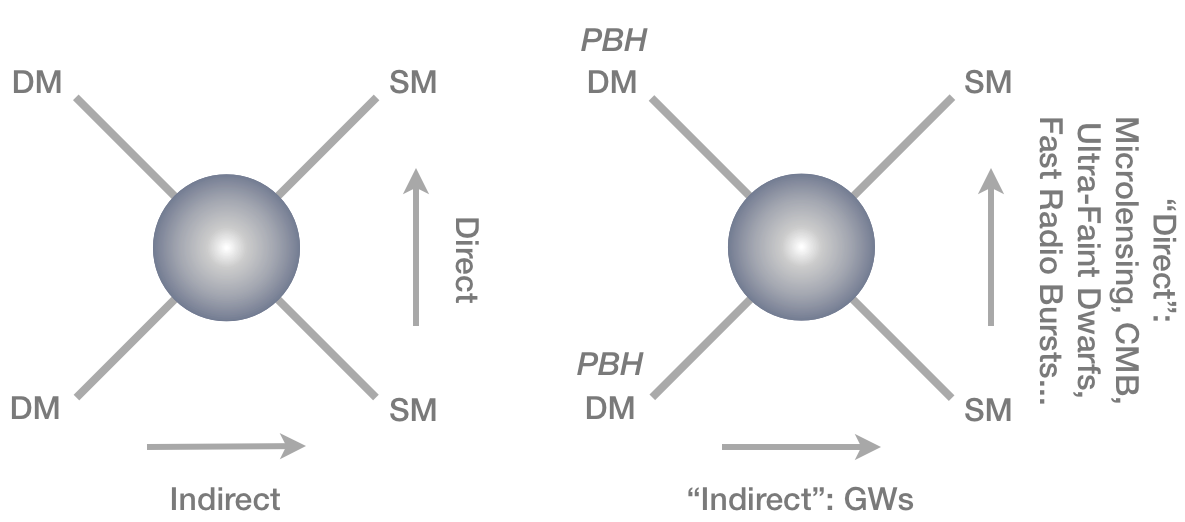
Indirect detection: As in the particle dark-matter case, the challenge is to quantify the standard model background and identify unique features of the desired signal. In Kovetz (PRL, 2017), I examined the indirect detection prospects with LIGO, taking into account the stellar-BH background. With JHU collaborators, we suggested to probe the progenitors of merging binaries based on the characteristically high orbital ellipticity in those formed by two-body capture. Finally, in recent work we considered the merger rate of early-Universe PBH binaries, estimating analytically the effects of interactions with baryons and other dark matter, and showed that LIGO may already place strong constraints on PBHs. Follow up work involves numerical simulations to verify this.
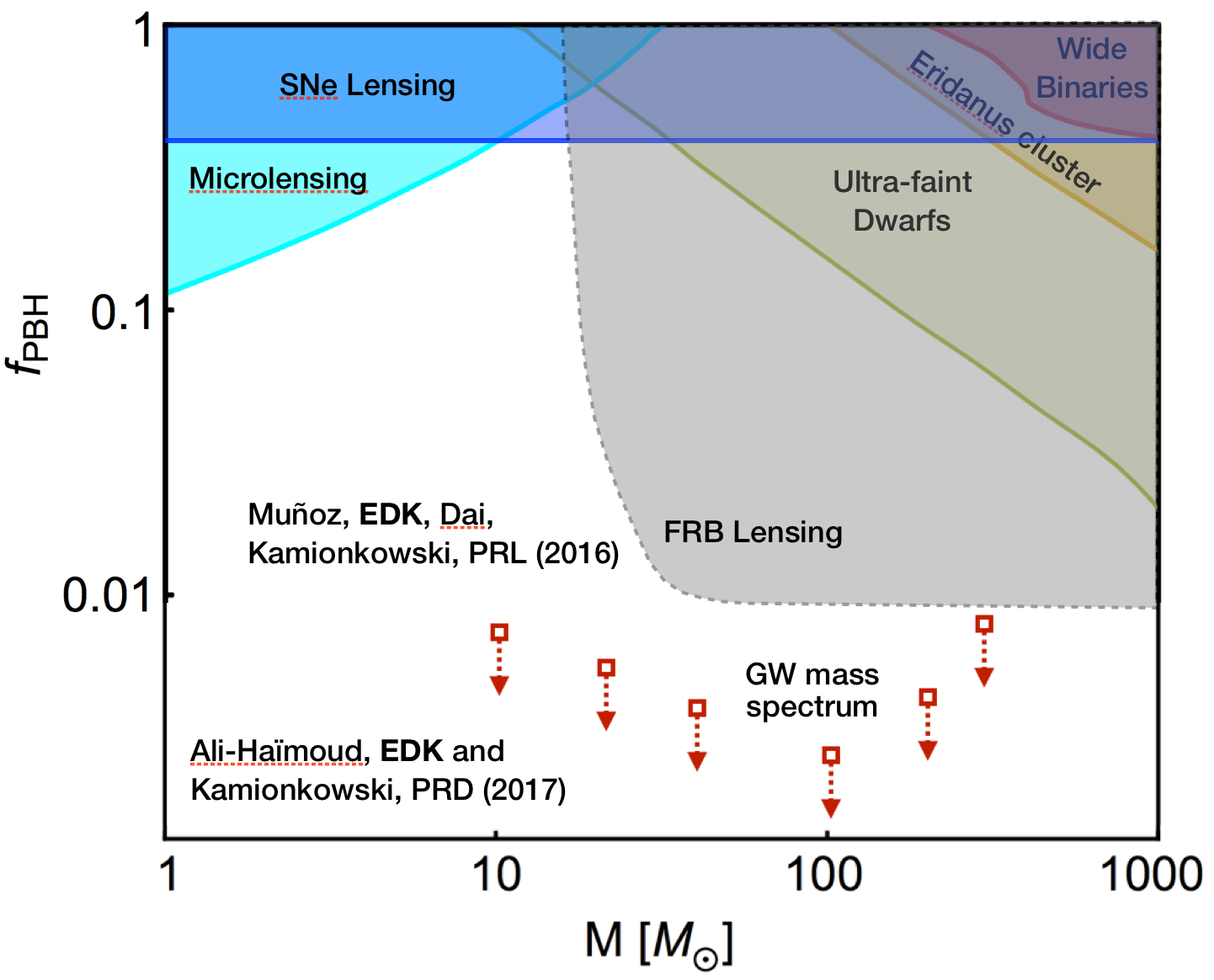
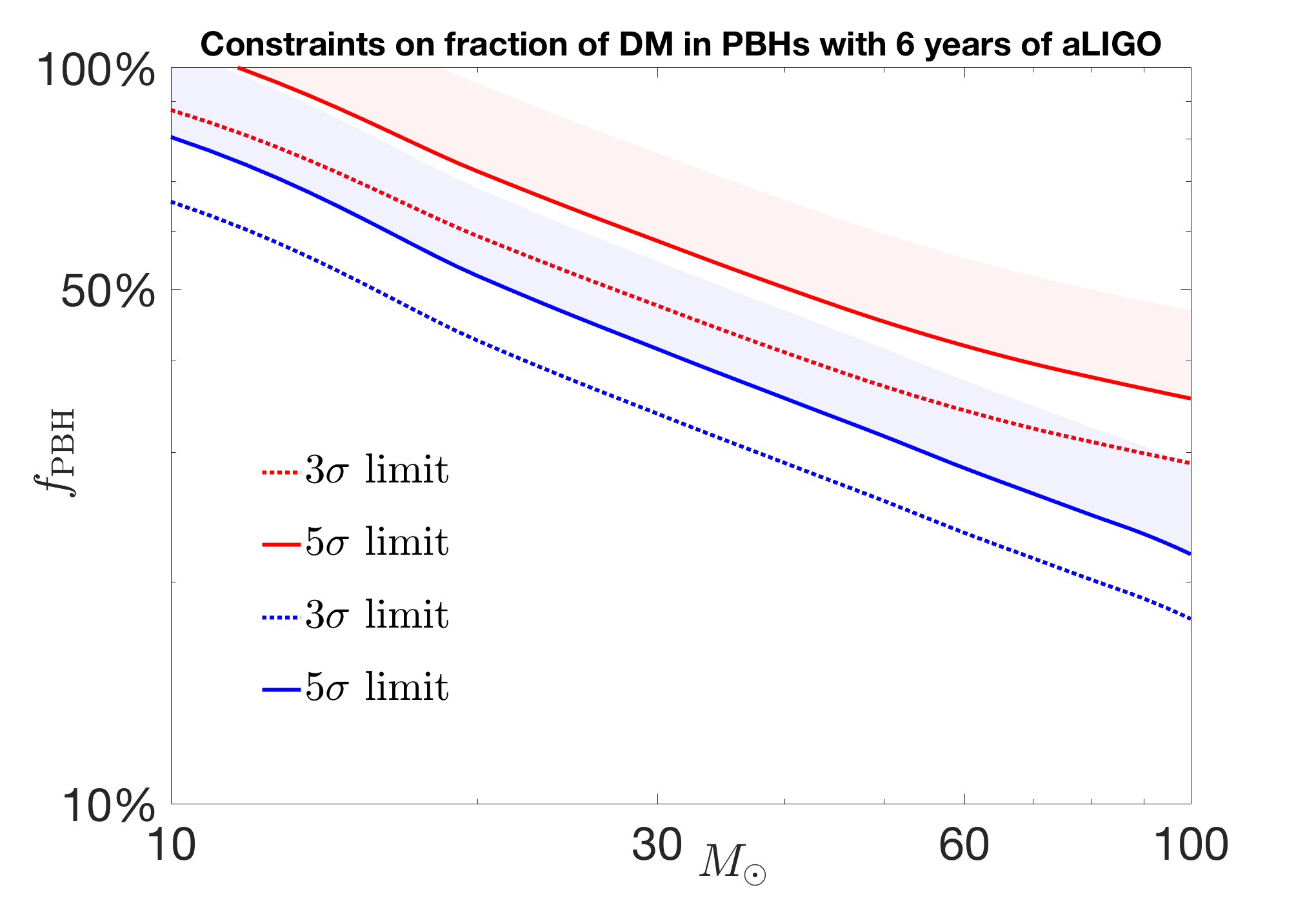
Right: Future constraints on the fraction of dark matter in PBHs, as a function of mass: Dashed lines correspond to 3σ limits, solid lines are more stringent 5σ limits. Constraints based on the 1D mass distribution shown in red; 2D distribution (tighter, as the noise per bin is smaller) in blue. For 30M⊙, we get fPBH 50% at 5σ (3σ) in the 2D (1D) case. Bands indicate factors 400% (200%) uncertainty in the background (signal) amplitude. From Kovetz (PRL, 2017).
- UPenn Astrophysics Seminar
(invited, 2017):
"Probing Primordial Black Hole Dark Matter”
(talks on this also given at APS2017/invited-session-talk, UW/Colloquium, UMN, Syracuse, WashU/Colloquium, Fermilab, BNL, USC/Colloquium, PASCOS2018) - Bird, Cholis, Muñoz, Ali-Haïmoud,
Kamionkowski, Kovetz, Raccanelli, Silk, PRL (2016):
"Did LIGO detect dark matter?"
- Raccanelli, Kovetz, Bird, Cholis
and Muñoz, PRD (2016):
"Determining the progenitors of merging black-hole binaries"
- Muñoz, Kovetz, Dai and
Kamionkowski, PRL (2016):
"Lensing of Fast Radio Bursts as a Probe of Compact Dark
Matter"
- Cholis, Kovetz, Ali-Haïmoud,
Bird, Kamionkowski, Muñoz and Raccanelli, PRD (2016):
"Orbital eccentricities in primordial black hole binaries"
- Kovetz, Cholis, Breysse and
Kamionkowski, PRD (2017):
"Black hole mass function from gravitational wave
measurements"
- Kovetz, PRL (2017):
"Probing Primordial Black Hole Dark Matter with Gravitational
Waves"
- Nishikawa, Kovetz, Kamionkowski
and Silk, submitted to PRD (2017):
"Primordial-black-hole mergers in dark-matter spikes"
- Ali-Haïmoud, Kovetz and
Kamionkowski, PRD (2017):
"The merger rate of primordial-black-hole binaries"
- Ji, Kovetz and Kamionkowski,
PRD (2018):
"Strong Lensing of Gamma Ray Bursts as a Probe of Compact Dark
Matter"
- Sato-Polito, Kovetz and Kamionkowski, PRD (2019):
"Constraints on the primordial curvature power spectrum from primordial black holes"
- Unal, Kovetz and Patil (2021):
"Multi-messenger probes of inflationary fluctuations and primordial black holes"
- Krochek and Kovetz, PRD (2022):
"Constraining Primordial Black Hole Dark Matter with CHIME Fast Radio Bursts"
Astrophysical Black Holes
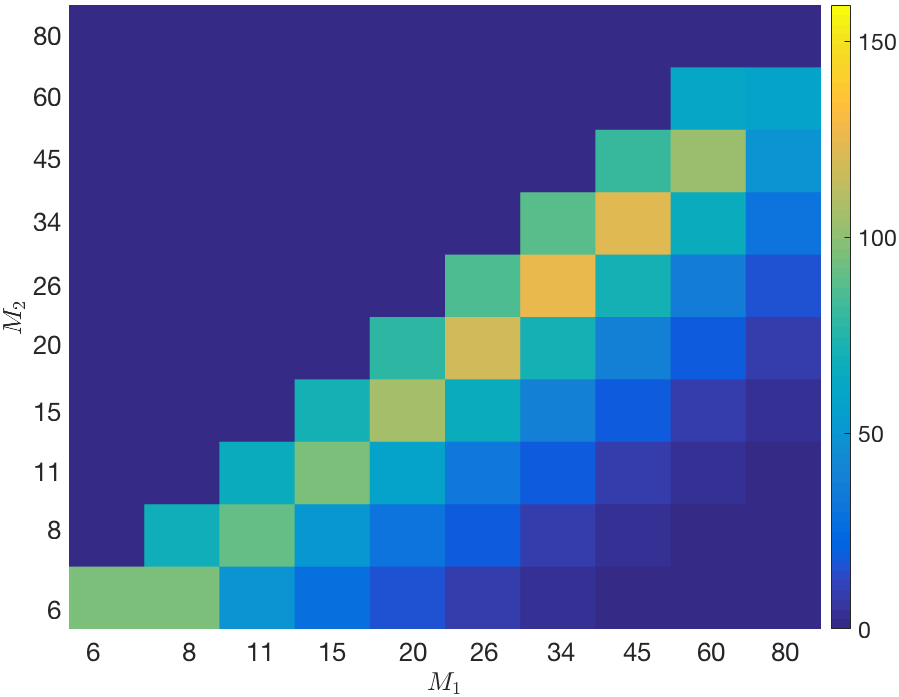
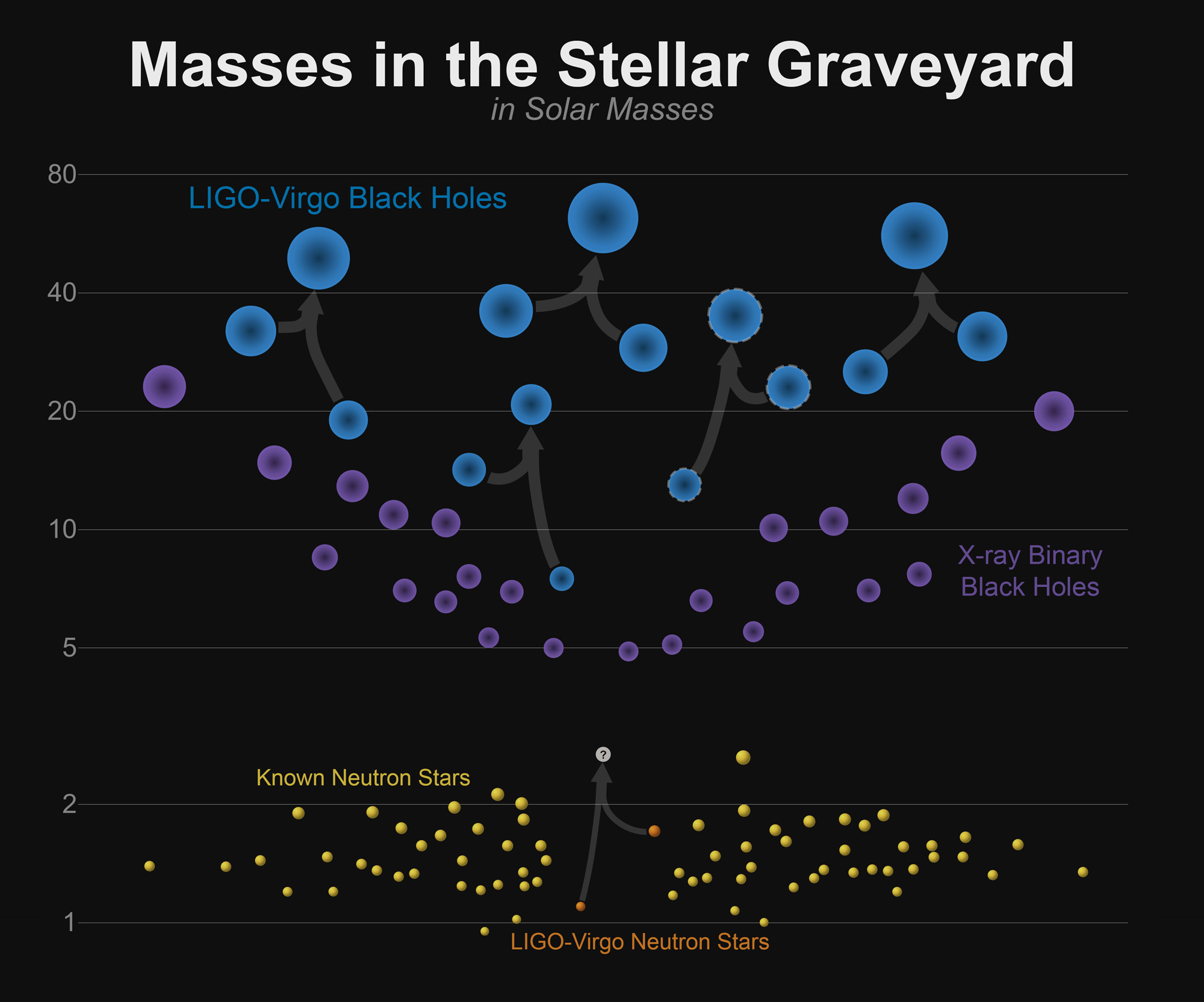
Right: LIGO-Virgo events, including mass gap between NS and BHs (Credit: LIGO/Virgo Collaboration).
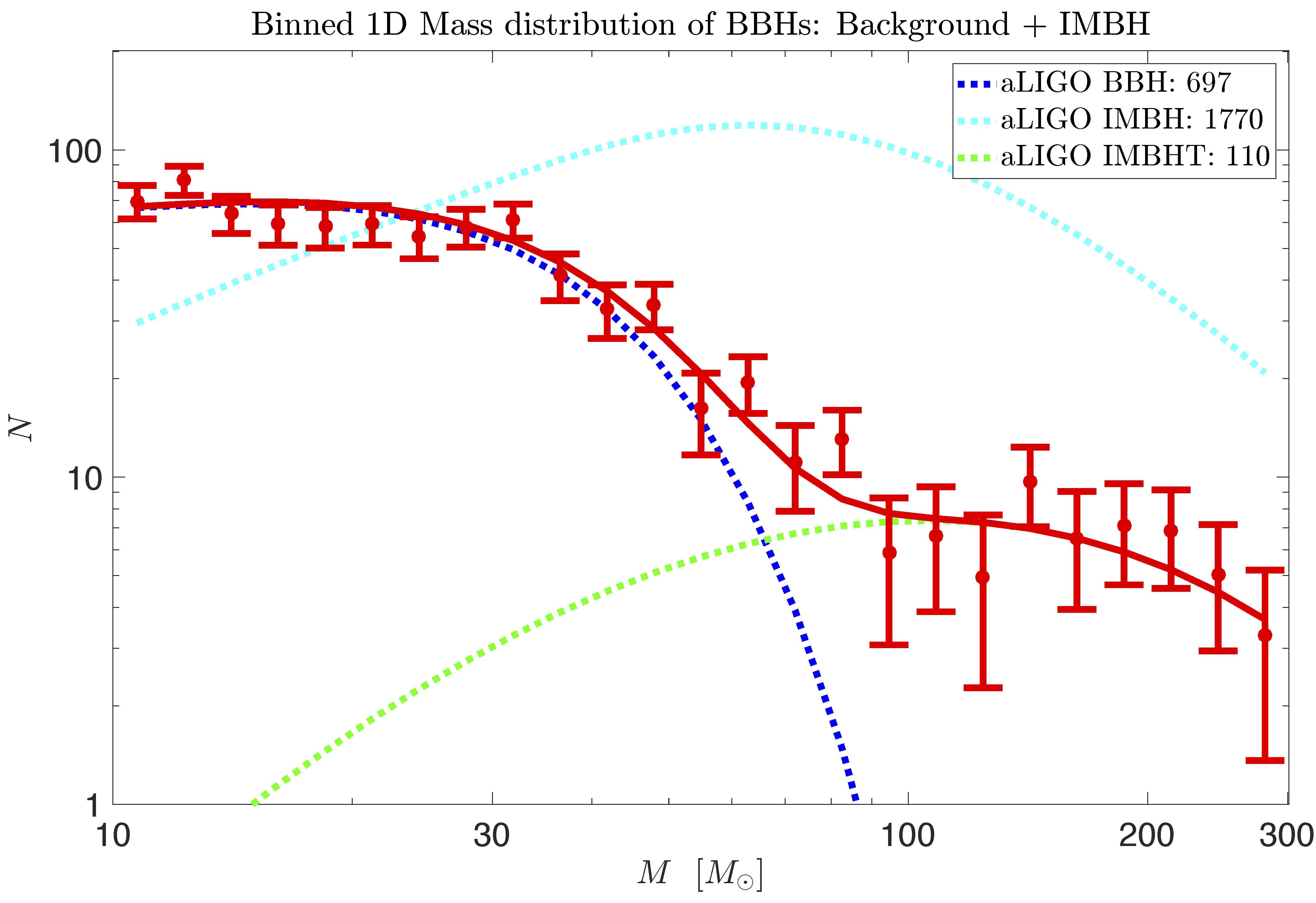
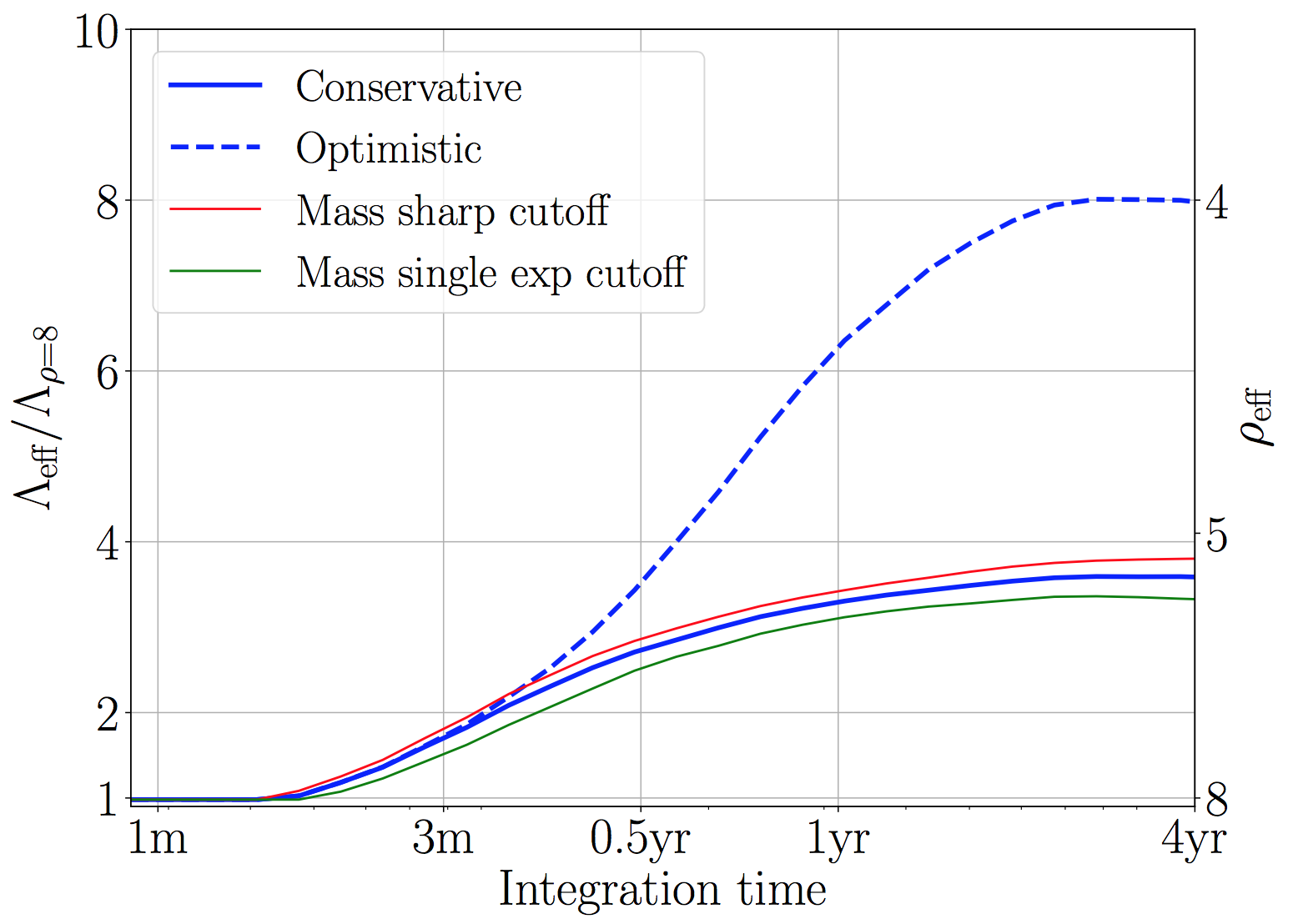
Right: Boost factor in LISA detection rate of stellar-mass BH mergers using ground-based information (Wong, Kovetz et al. 2018)
- Tel-Aviv University Astro Seminar (invited, 2016): "Gravitational Waves from Merging Black Holes as a Probe of Astrophysics and Cosmology”
- Kovetz, Cholis, Breysse and
Kamionkowski, PRD (2017):
"The Black Hole Mass Function from Gravitational Wave
Measurements"
- Kovetz, Cholis, Kamionkowski and Silk, PRD (2018):
"Limits on Runaway Growth of Intermediate Mass Black Holes
from Advanced LIGO"
- Wong, Kovetz, Cutler and Berti, PRL (2018):
"Expanding the LISA Horizon from the Ground"
- Flitter, Muñoz and Kovetz, MNRAS (2021):
"Outliers in the LIGO Black Hole Mass Function from Coagulation in Dense Clusters"