Sample projets (outdated):
(back to research highlights)
|
| Self similar ultra-relativistic jet solution |
|
| Analytic solutions for hydrodynamic and magnetohydrodynamic flows |
|
| Core collapse supernovae |
|
|
Radio halos and relics
|
|
|
Spiral flows in galaxy clusters |
|
|
Cold fronts in galaxy
clusters |
|
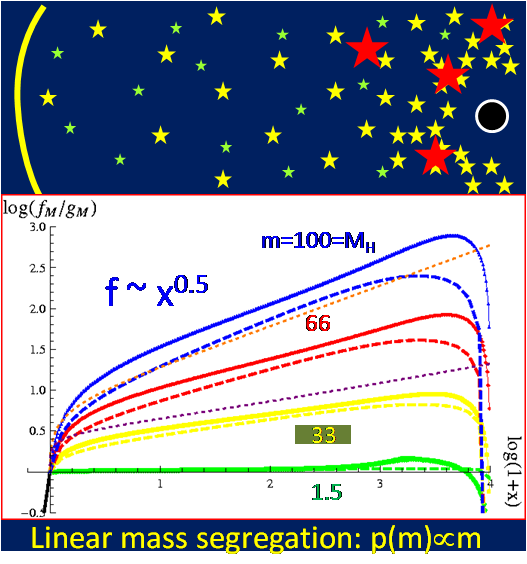
|
Bahcall & Wolf (1976,1977)
have shown that within the radius of influence of a massive black hole,
stars of different masses segregate, each species forming a power-law
density cusp. The upper left image illustrates mass segregation of
stars (light stars in green, intermediate mass-yellow, and massive-red)
around a massive black hole (black disk). We derived an analytic
solution for the energy distribution f(x,m) of stars with an arbitrary
mass function g(m) (illustrated in bottom left image). At intermediate
distances f~x^p, where p(m)=m/4M and M is an averaged stellar mass (see details). The right
panel shows the landscape of the energy power-law indices pH of the
most
massive stars, for different power-law mass functions
g(mL<m<mH)~m^α,
as a function of power-law index α and the mass range ζ=mH/mL. |
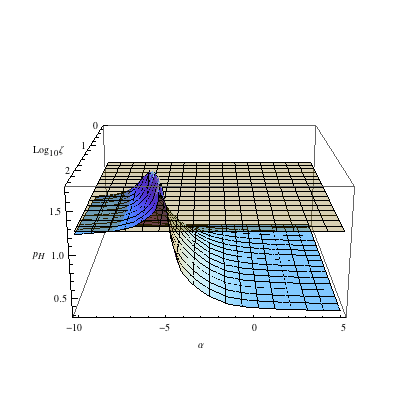
|
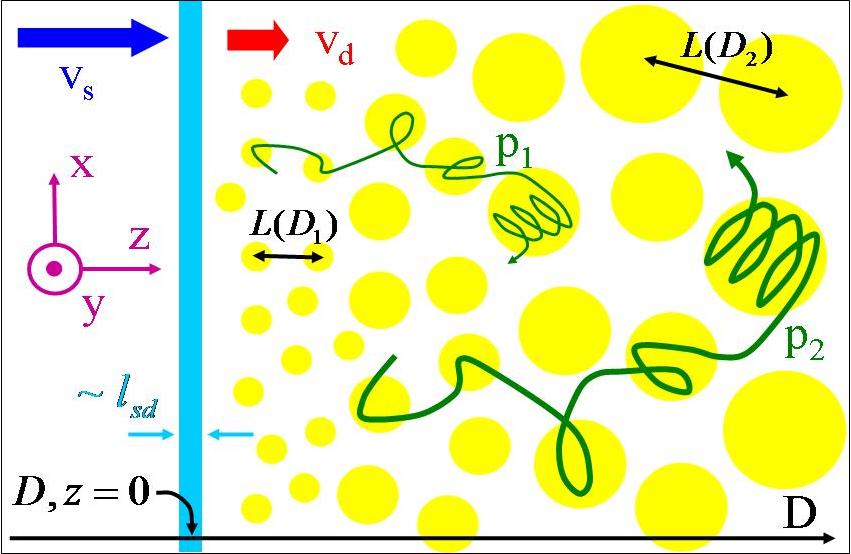
|
Modelling
a collisionless shock requires a self-consistent treatment of the
electromagnetic field and the plasma, including the high energy tail
which plays an important role in strong shocks. Motivated by γ-ray
burst observations, we
derived
the scalings of self-similar solutions where the plasma is
approximated as a combination of kinetic and MHD components (left:
cartoon of downstream structure).
Numerical simulations, notably particle in cell (PIC), are gradually
probing more
advanced stages in the evolution of shocks. We used ab-initio PIC simulations
to demonstrate that the particle distributCCCion and the magnetic field
evolve together over long time scales, not probed previously. The image
on the right shows the spatial structure (in the shock frame; upstream
is to the right) of a shock at early (panel a; blue curves) and late
(panel b; red curves) times. |
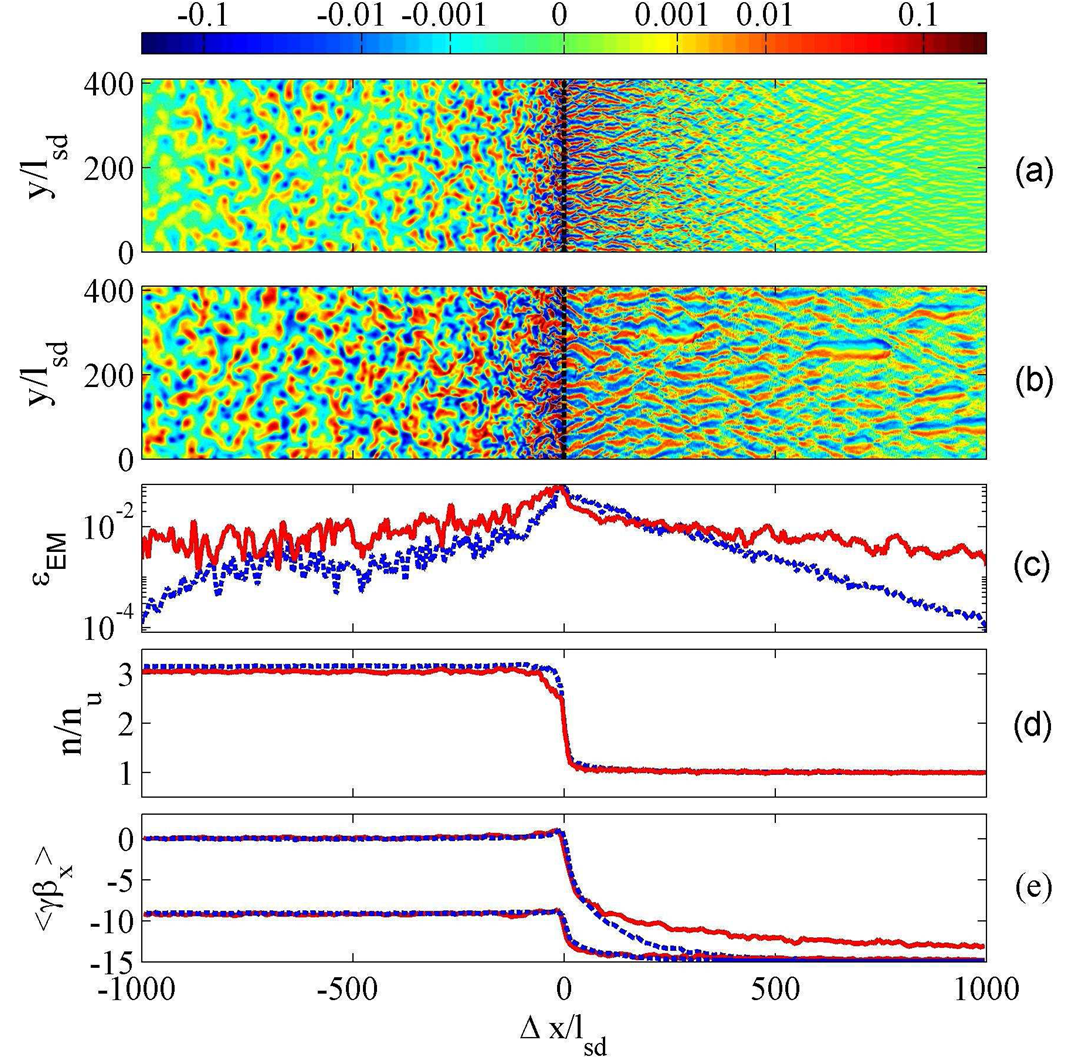 |
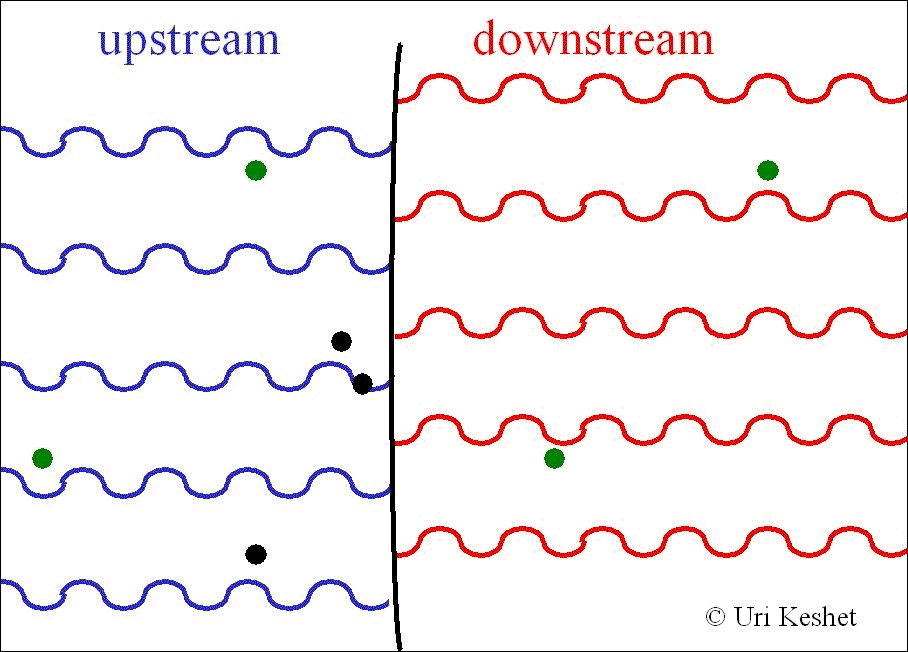 |
Collisionless shocks are thought
to Fermi
accelerate
a power-law spectrum of charged particles by repeatedly scattering them
across the shock front
(left: illustrating one Fermi cycle). For isotropic diffusion the
momentum spectral index was found to be s=(3βu-2βuβd2+βu3)/(βu-βd),
where β is the shock frame fluid velocity normalized to the speed of
light (right panel: s for different equations of state). For
ultrarelativistic shocks this gives s=38/9, in agreement with GRB
afterglows. However, for relativistic shocks s is sensitive to the
diffusion function, especially downstream. |
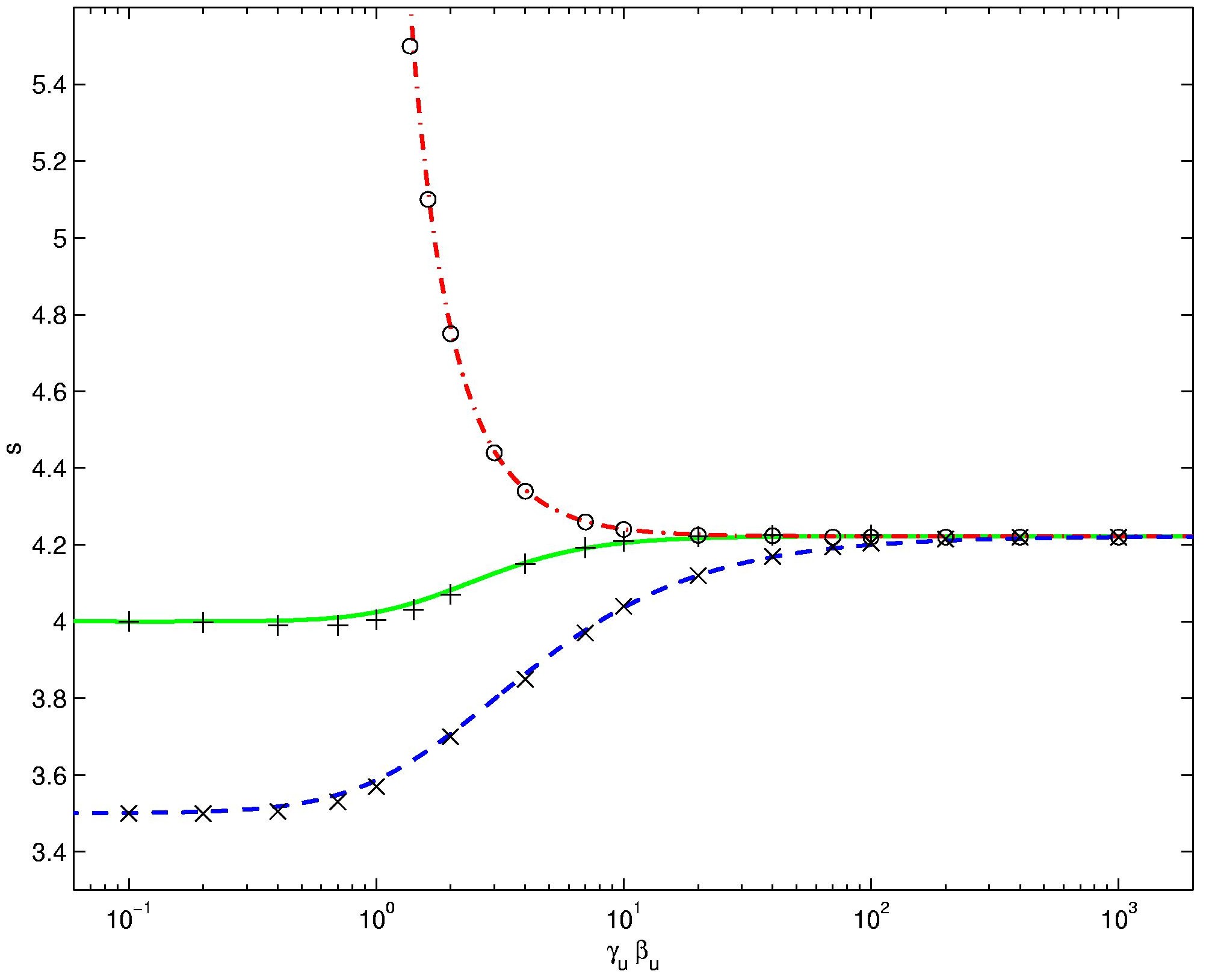 |
|
Black
holes have characteristic spectra of ringing (quasinormal) modes
characterizing their perturbations, manifest for example as
gravitational waves emitted in the last stages of black hole merger
(left image: LISA artist
impression). These and related
resonances depend only on black hole parameters (mass, rotation and
charge) and general relativity - no equation of state is involved. They
share some similarities with atomic spectra (e.g. possible selection rules). The intermediate and asymptotic (right
image) quasinormal spectrum and greybody factors of a
general (Kerr-Newman) black hole were analytically derived [1, 2 ,3], providing clues on
quantum gravity. |
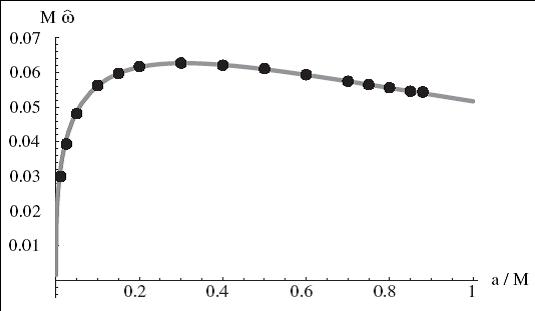
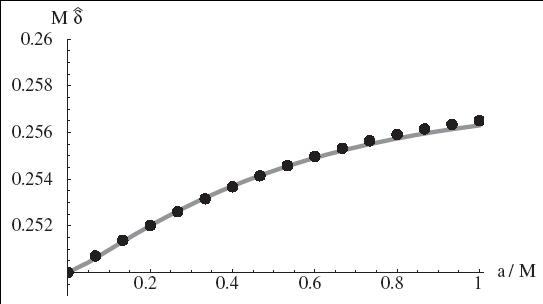 |
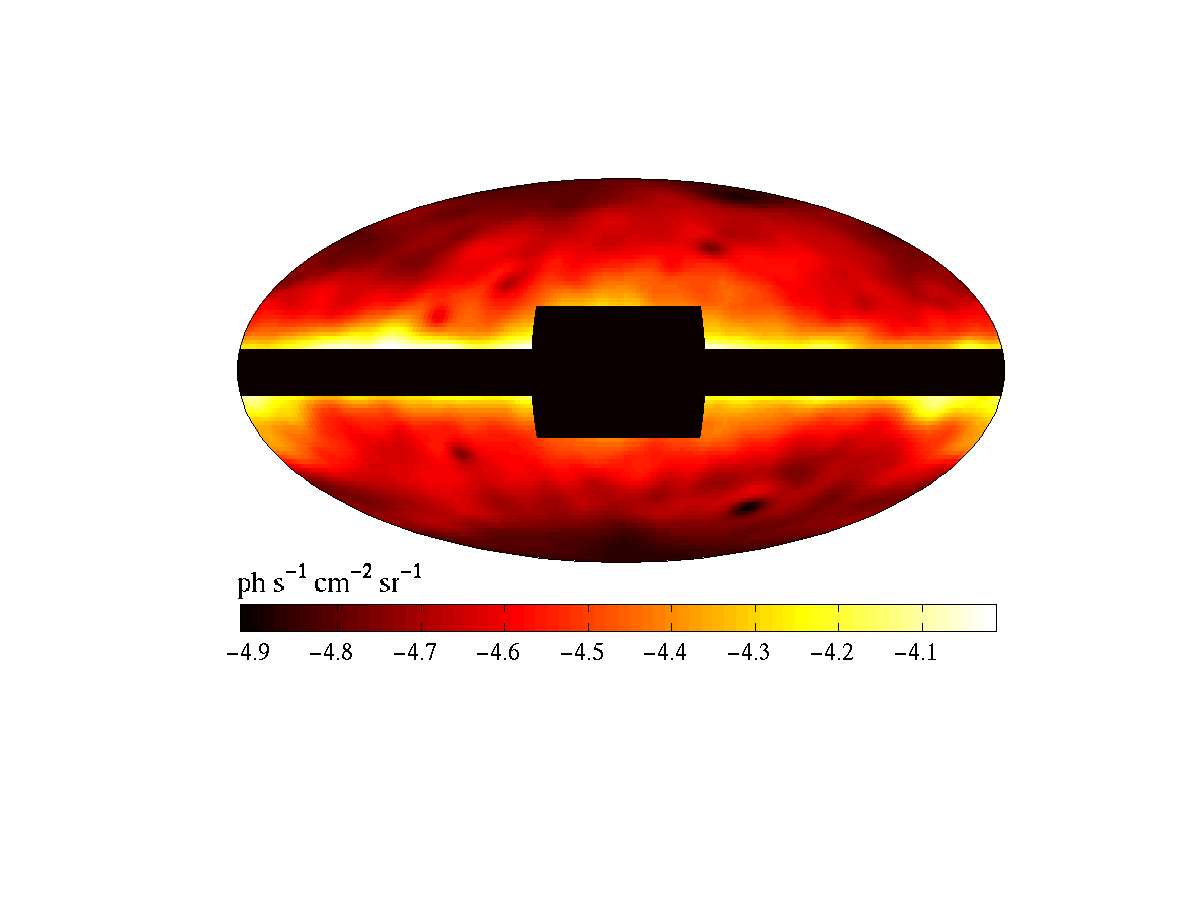 |
The diffuse extragalactic
backgrounds in γ-rays
and in <1GHz
radio
frequencies are not well-known. The γ-ray sky (left image,
from EGRET) shares several features with tracers of our own Galaxy even
at high Galactic latitudes
(e.g., Hα emission, right image), suggesting that
the diffuse emission originates mostly from
cosmic-ray interactions
within the Milky-Way [credit]. |
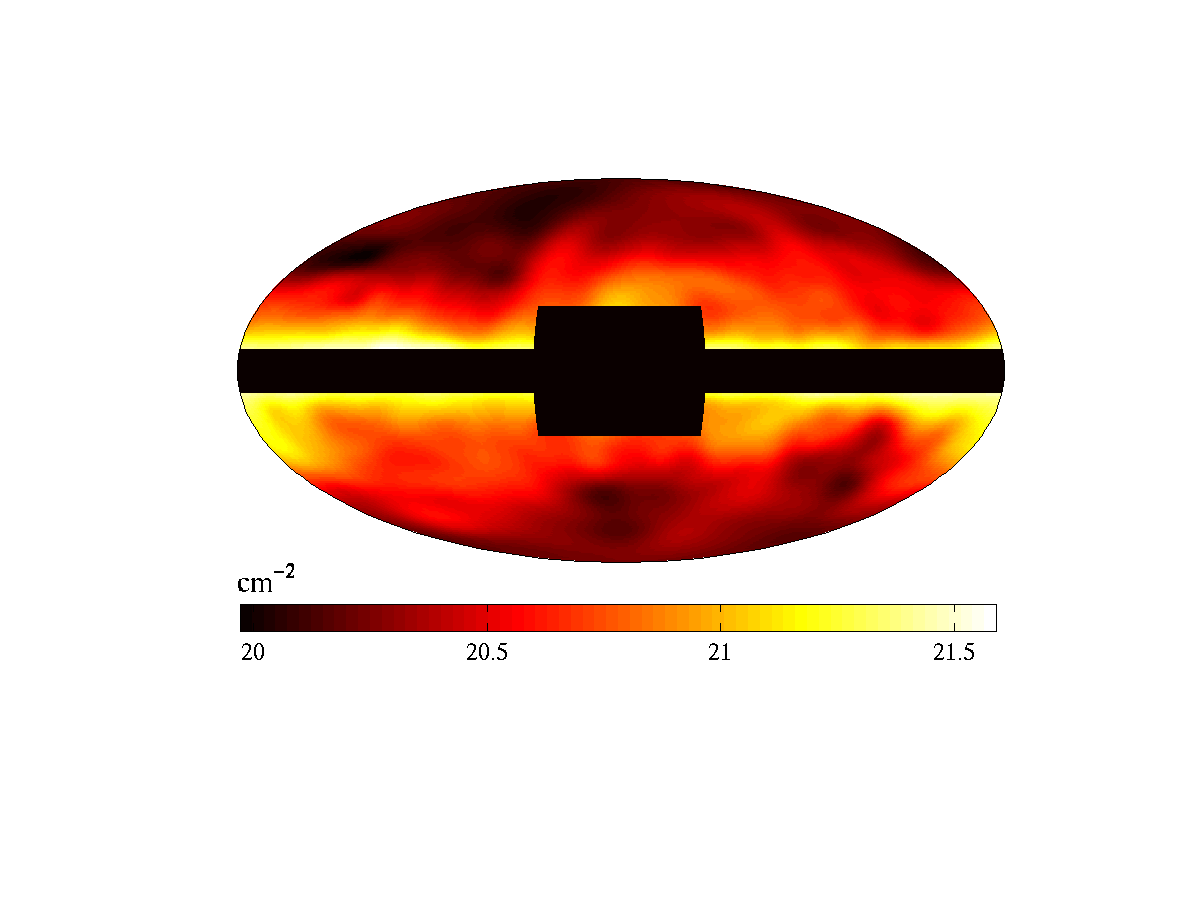 |
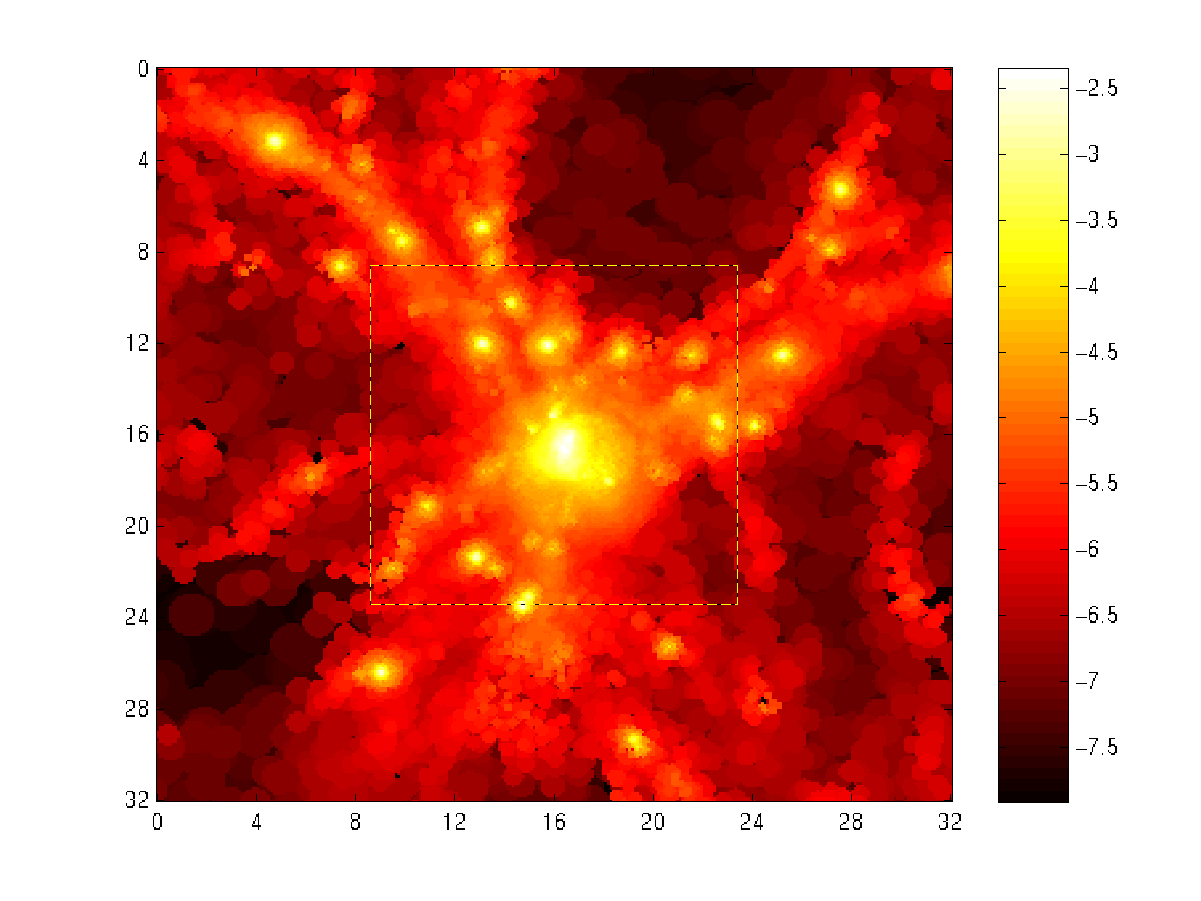 |
Large
scale accretion shock waves are an inevitable outcome of structure
formation in the Universe. These shocks should be observable
in γ-rays by the 5-year Fermi
mission [1, 2] and in radio [1, 2] by the SKA possible by LOFAR. Preliminary
observations in both γ-rays and radio have been
reported.
Images: simulated galaxy cluster (left: baryon number
density) and
associated γ-ray emission (right: depicting observed image of the
region marked on left) [credit].
(Shocks also occur as galaxy or galaxy
clusters collide, see e.g. Stephan's quintet in radio,
infrared and x-rays.) |
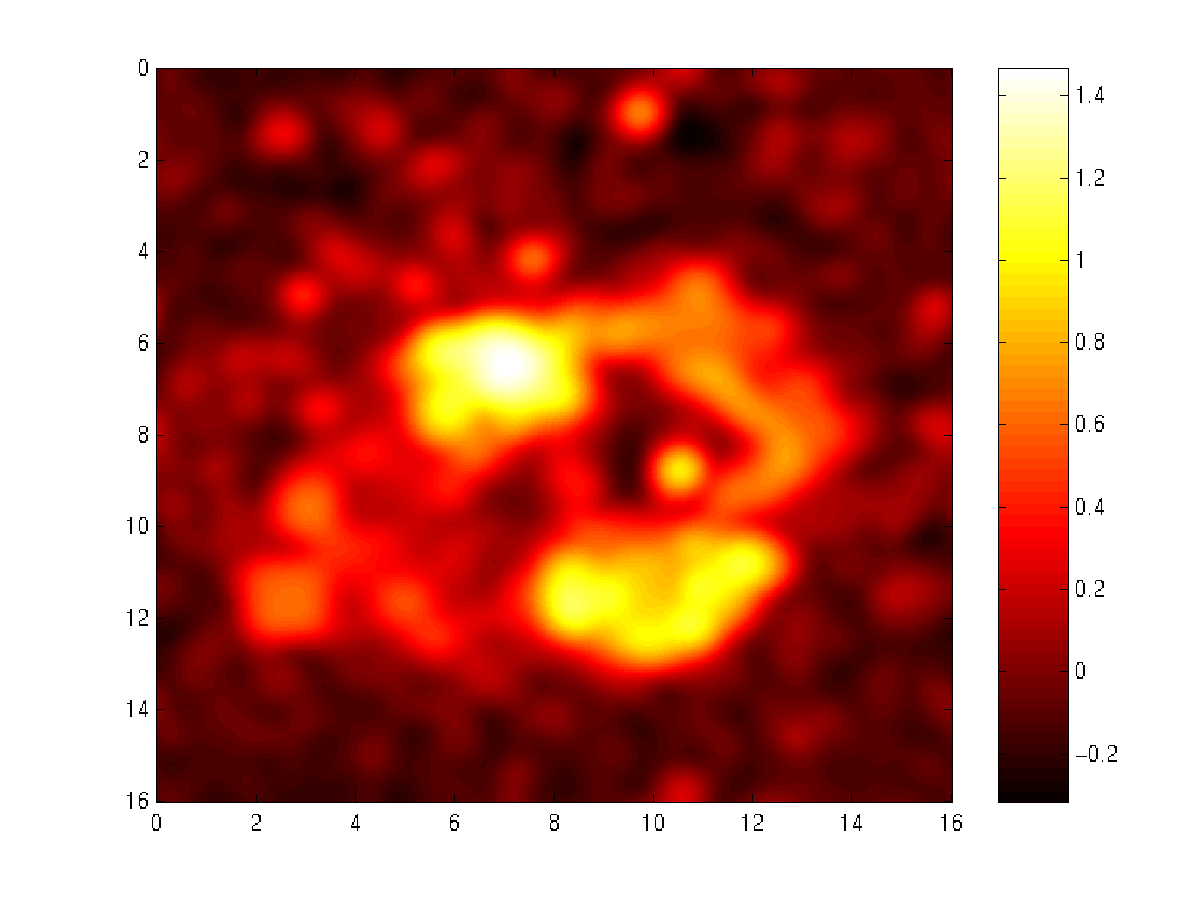 |
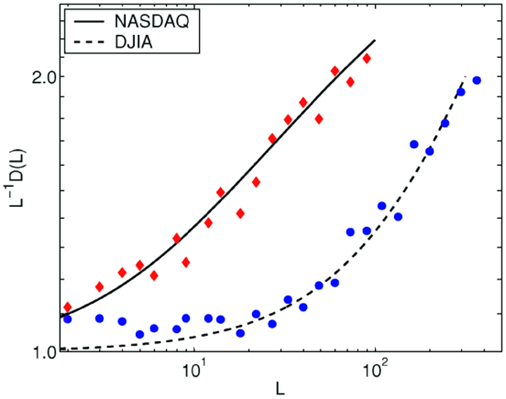
|
A wide variety of
dynamical systems with long-range correlations can be analyzed in terms
of history-dependent random walks. We presented a simple,
analytically solvable model for random processes with long-term memory,
which exhibits a dynamical phase transition from normal to
super-diffusion. The model can be used, for example, to quantify stock
market behavior (left plot). A related phase transition from finite to
power-law decaying survival probability S(L) is found in the presence
of an absorbing barrier (right plot). This describes, for example, an
investor's probability to remain in profit at time L.
|
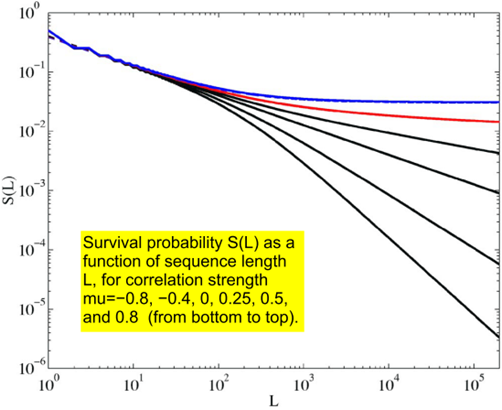
|